GIFs in the Genome: Francis Crick, Eadweard Muybridge, and the Photographic Frame
In February 1953, Francis Crick rose into the pantheon of the great 20th century scientists. Together with his colleague James Watson, he proposed that the double helix, that elegant intertwined spiral, was the fundamental shape of DNA. Aside from being a triumph of structural biology, it formed the basis for answering elemental biological questions about DNA mechanics: how is it accurately replicated? How does it archive information? How is it read? Simply: base pairing. Adenine pairs with thymidine and cytosine with guanidine; these four bases are enough to encode a stable language that directs the cell to invent itself.
In unveiling the iconic structure of the double helix, Francis Crick helped to establish the central dogma of genetics, which defines an ineluctable and unidirectional flow of information from DNA, to RNA, to protein. Crick’s dogma defined DNA as the master template, the prime mover. Today, with biotechnologies exponentially increasing our ability not only to observe but also to manipulate our DNA, we subvert the central dogma, with information flowing, at our discretion, into DNA. The instinct to control nature as men of rationality is not new, but as scientific wizardry reaches so far as to fiddle with our own genetic makeup, it becomes necessary to reexamine the impulse.
The wizardry du jour is CRISPR (clustered regular interspaced short palindromic repeats). A biological curiosity in its own right, CRISPR technology is derived from a mechanism used by bacteria as a sort of proto-immune system. When invaded by a virus, the bacterial CRISPR machinery inserts bits of the viral DNA into a special location in its own genome called an “array,” dynamically recording its history of infection. The next time a virus invades, the bacteria can draw upon its molecular memory to recognize the virus and mount a counterattack. Researchers quickly realized, though, that CRISPR could be tricked into inserting any DNA into the genome of nearly any organism—including humans. Laboratories sprang to demonstrate both proof-of-concept and practical applications from the whimsical to the ethically dubious (the rumored Chinese CRISPR babies come to mind).
A recent effort by the Church lab, a synthetic biology group at Harvard, used CRISPR to encode visual information into living bacteria and then accurately recover the image generations later. Because the bacteria incorporate the foreign materials linearly in time and in space, the researchers hypothesized that by staggering introduction of the DNA, they could in effect record film into the bacterial genome. The biology of the CRISPR array—in which each viral sequence, called a spacer, is separated by short areas of repetitive DNA sequence—indeed conjures a film reel, with each snapshot in time separated by a frame that delineates its boundaries while linking it to the next.
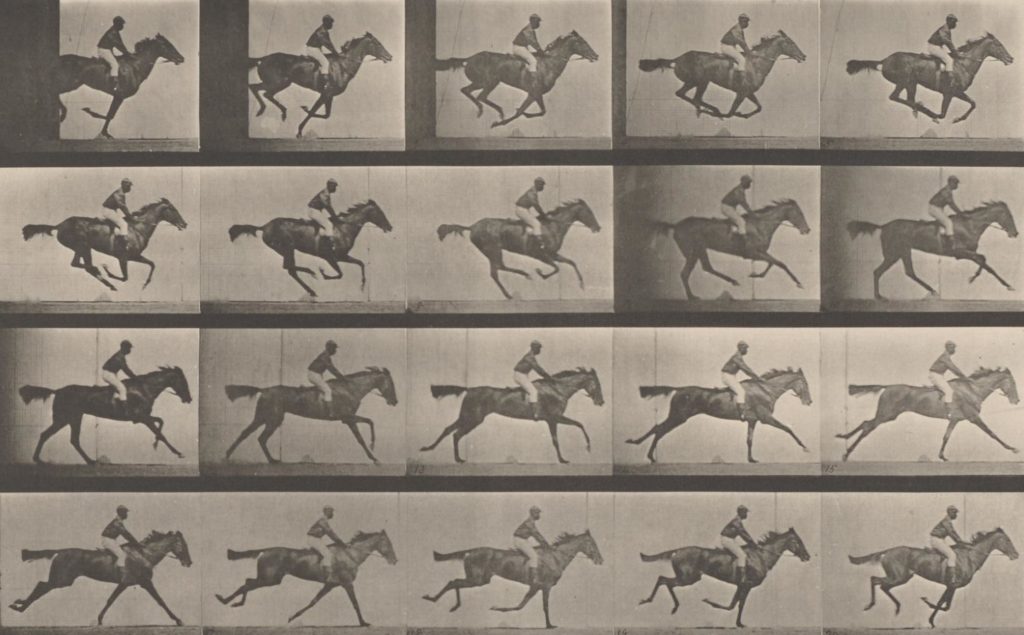
Eadweard Muybridge, “Annie G.” Galloping, Plate 627 from Animal Locomotion: An Electro Photographic Investigation of Consecutive Phases of Animal Movements, Vol. 9, Collotype, 1887. Image via the Philadelphia Museum of Art.
The research group chose for their subject a few frames of an Eadweard Muybridge galloping horse. Asked by The Atlantic why they chose it, lead author Seth Shipman explains, “it was one of the first examples of a moving image, it was captured with a technology that was very new at the time, and it answered some relevant questions.”[1] I’d go a step further: encoding this image is a parallel instance of capturing natural phenomena in the pursuit of technological progress. Muybridge was hired to use his imaging technologies to deconstruct the gallop by a prominent tycoon, Leland Stanford, who wanted to use gait data to improve his horse breeding program. The horse, a mare named Annie G, has now been pressed into the service of bioengineering twice over, doubly fixed in her perpetual gallop by men of science.
Muybridge’s images were originally captured on a zoopraxiscope, an instrument he developed to animate a series of still images and one of the earliest versions of motion pictures. Like in the CRISPR experiments, Muybridge depended on a unidirectional timescale: if we can control the order of events, we can reconstruct them into a logical sequence. In this way, isolated snapshots in time are converted by the brain into perceived motion, with the motion itself existing ineffably between the frames. Muybridge’s photographs superseded the capacity of the human eye to see events; they placed scientific order above experienced perception.
In the CRISPR experiments, the clarity of the recovered image is dependent not on camera technique but on brute-force DNA sequencing: leveraging technological power against natural lacunae (for example, based on their nucleotide makeup, some sequences are significantly less likely to be incorporated into the CRISPR array than others). To reduce the overall volume of data that must be collected—to recover even this amount of data with high accuracy, the researchers needed to analyze over four million individual sequences—the image had to be adapted into a heavily pixilated version, blurring Muybridge’s expansive vision. The bunching muscles of the horse are reduced into blocky binary, but the hypnotic motion remains.
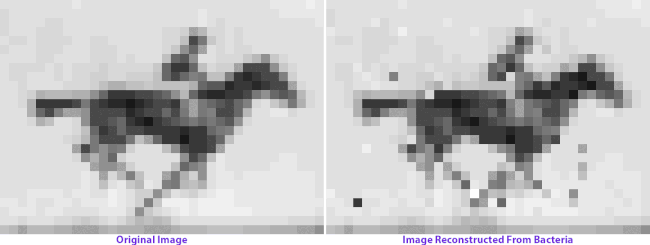
On the left: frames from Eadweard Muybridge’s Human and Animal Locomotion. On the right: frames after multiple generations of bacterial growth, recovered by sequencing bacterial genomes. Images courtesy of Seth Shipman.
How the image encoding basically works is that each nucleotide triplet represents a pixel gray value, and each piece of targeted DNA contains the code for 9 pixels plus information about where they’re located in the grid. The full grid is encoded by 104 total pieces of DNA, or oligonucleotides. Each cell will only incorporate a small subset of the oligonucleotides into their CRISPR array, and the image can only be reconstructed by averaging the information from a vast population. A computer program ranks the most frequent pixel sequence associated with each designated location, and applies iterated “ordering rules” to orient them in the grid: Muybridge’s organizational principles magnified by orders of magnitude.
Fixation
Both Crick and Muybridge sought to capture an essential natural architecture. Crick hunted DNA structure and Muybridge looked for anatomical structure—the gait of the horse, the throwing of a punch, the unfurling of a girl’s arm as she rises from a crouch. It is critical that both achieved their goals by stilling the natural world. The X-ray crystallography technique that helped decipher the double helix used DNA that was quite literally frozen in place (crystallography comes from the Greek crystallon, or “frozen drop”); the National Museum of American History is currently hosting an exhibition of Muybridge’s work called Freeze Frame. I think of scientists entombing their samples in the hulking minus-80 degree freezers, or the racks submerged in foggy liquid nitrogen, so cold your fingers go numb and burn even under two pairs of gloves, temperatures low enough to arrest biological processes and hold entropy at bay.
My imperfect understanding of Heisenberg is that to observe is to alter, and while the quantum ghosting of the physics doesn’t apply to larger objects, like DNA or, say, an entire horse, it is true in many cases that in order to observe something you must affix it somehow, in time or in space. We rarely see the infinite, stochastic teemingness of life, only snapshots. To harness nature is to break it, in some rude capacity, like a broken horse tamed to the bit. Like the zoopraxiscope, we only animate our hypotheses in post-production.
The violence inherent in fixation stretches throughout natural history. Before Darwin developed his sweeping view of the origin of species, he catalogued species, capturing the finches’ beaks in barry graphite or in specimen bags. The legendary ornithologist John James Audubon, whose legacy is famously conservation, slaughtered thousands of native birds in order to obtain more static models for his drawings. Muybridge’s photographic series cage his subjects on the page, each individual image graphed against charts and grids, separated frame by frame, reduced to parts. Personally, I’ve never murdered a passerine, but I’m well familiar with the funerary formaldehyde reek of cell collection.
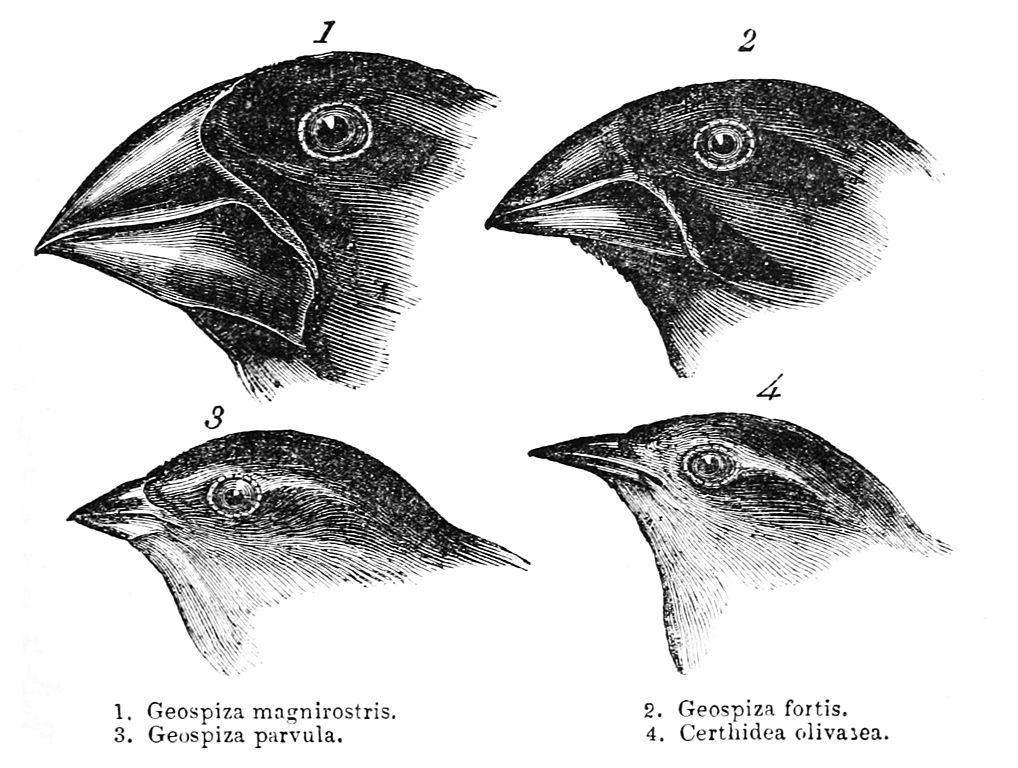
Charles Darwin, Darwin’s finches or Galapagos finches, from Journal of researches into the natural history and geology of the countries visited during the voyage of H.M.S. Beagle round the world, under the Command of Capt. Fitz Roy, R.N. 2nd edition, original made in 1845. Image via Wikimedia Commons.
Distilling a flying finch down to a flat image, to a structure, a theory, is a diminution of its quiddity. What happens when the subject is human? Anthropological photography, biology, and medicine all have taproots deep into human injustice and ethical shadow. When Muybridge turned his lens from horses to the human corpus, he racialized the camera’s gaze. The photograph became a vehicle not only to catalog the human body but also to contextualize physical and moral meaning onto it. Anthropological and vernacular photography of the era offered a communal data archive that could be used to support the belief not only that physiology informed destiny,[2] but also that the Black or native body was naturally other, which is to say inferior, sorry! it’s just science.
When a human body is so coolly cataloged in print and phenotype, it ignores our shared humanity and, rather, sees the person as object. Once personhood is stripped from the body, so follows agency. The legacy of using Black bodies for medical research without their consent—J. Marion Sims performing gynecological research on enslaved women, the Tuskegee syphilis experiment, and the HeLa cell line biopsied from Henrietta Lacks’ tumor being the most prominent examples—can be linked to the same dehumanization.
Our stable of geneticists doesn’t fare much better. Crick privately expressed belief that eugenic solutions could improve the human race; for example, that wealthy parents should be encouraged to have more children (given what we know about socioeconomic demographics, the word wealthy is doing a lot of work here). His partner, James Watson, was recently stripped of a number of honors due to a galling and unapologetic statement that he believes (current tense!) that people of African descent are less intelligent than whites by genetic determination. Once again, dehumanization stems from science’s instinct to identify an evaluable quantity and impose external order onto a complex system.
Creation
DNA imparts identity as a source of metaphysical continuity. If, like the Ship of Theseus, all of our cells are eventually replaced by new cells due to turnover (this is not strictly true, but we can certainly consider ourselves to be inconstant beings), can we be considered the same person? With sequence as self, DNA can serve as a thread stitching past-yous to future-yous like different frames in a coherent movie, linked by faith and mental infill.
It’s either reductive or absurd, depending where you fall along the woo spectrum, to conflate genetic uniqueness with some higher animating principle, but it’s not without precedent. One’s self-image, no less than a molecular signature, can confer identity. And after all, some cultures believed that act of being photographed took a piece of the soul, referring to photographers as shadow catchers, trapping a ghost-layer on the photographic plate. Science bioethicists debating embryonic stem cells or human genome editing find the argument, in the end, distilled to fundamental questions about duality versus psychosomatic unity. But as science becomes increasingly quantitative and logic-driven, questions of the soul are often put aside. Today, we snap a front-camera-ed selfie as carelessly as we dribble into a tube to ship off to 23andme and receive our fate (or at least learn our type of earwax).
It is therefore unsurprising that, in parallel with our exploration of the relationship between genetics and self, researchers have also been stripping DNA of its metaphysics and converting it into a tool of pure information. DNA has, in the past, been used to: encode and store the entirety of War and Peace,[3] help solve the traveling salesman problem,[4] and encrypt secret information in scenarios straight out of a spy novel. (That last technique, called DNA steganography, recaptures its organic roots: when the Greek Tyrant Histiaeus wanted to send a secret message, he shaved the head of a slave, tattooed the information on his pate and waited for the hair to grow back in before sending him off to get shorn by the message’s recipient.) So what makes DNA in a tube distinct from DNA in a living organism?
Mutation
The answer, I think, is that DNA in an organism is still dynamic. Indeed, storing data in the DNA of living organisms (as was demonstrated in the Church lab’s work) compared to DNA freeze-dried in a plastic tube, is limited by its mutability. Each day, naturally occurring DNA damage inflicts approximately 70,000 lesions per cell, and the depredations of ultraviolet radiation, chemical mutagens, and other external sources of damage only increase the cell’s burden. To counteract this extraordinary assault on genome integrity, cells have evolved a deep bench of DNA repair mechanisms. Crick himself, twenty-one years after discovering the double helix, mused “We totally missed the possible role of enzymes in repair although I later came to realize that DNA is so precious that probably many distinct mechanisms would exist. Nowadays, one could hardly discuss mutation without considering repair at the same time.”[5]
DNA repair proteins are like the librarians of the genome, bulwark against Babel. They mend broken spines, replacing nucleotides that have been warped by bulky adducts like books left in the rain. When they find gaps, they can refer back to another edition of the genome (the sister chromatid) and restore it word-for-word. I always found it comforting that DNA encodes the tools it needs to fix itself.
Working as a DNA repair biologist, I would subject my cell cultures to the veriest of tortures, strewing their food with chemotherapy drugs, taking them to the cesium irradiator (a source of gamma rays—the type of radiation emitted during a nuclear explosion—that was unsettlingly located in a seemingly-random hall closet in the basement). It wasn’t personal, most of the time, but sometimes the cells I cheerfully tormented in the pursuit of scientific knowledge were HeLa cells.
In vitro human studies depend upon the maintenance of established cell lines derived from human tissues and made immortal; that is, they have naturally achieved or were manipulated into evading the natural Hayflick limit on how many times a cell can divide. All the cell lines have names (even as a grown woman, I snickered that the cell line dubbed BJ was derived from foreskin fibroblasts), and what almost began to feel like personalities. These ones don’t like being left at room temperature for too long, these other ones are jerks about adhering to coverslips. Only one cell line has a face.
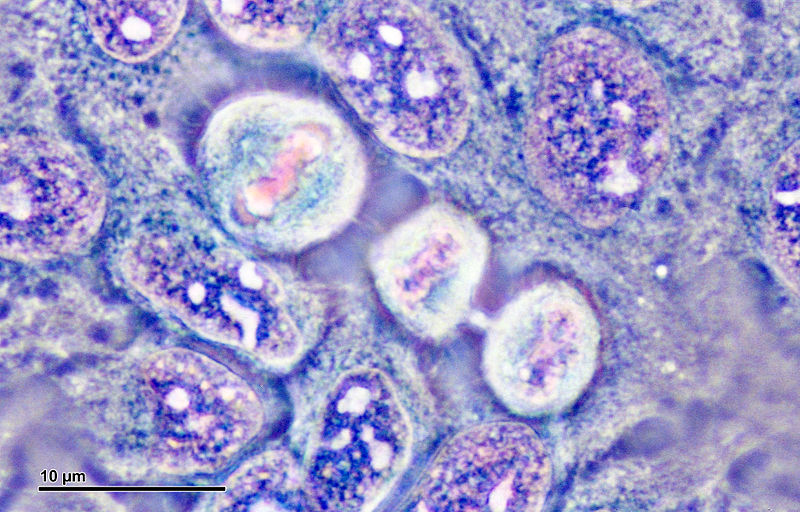
Dividing HeLa cell cultures. Image via Wikimedia Commons. [CC BY SA 3.0]
Henrietta Lacks, the unknowing and non-consenting donor of the workhorse HeLa cell line, was a poor black woman diagnosed with cervical cancer. Because her cancer cells had become immortal, they could be cultured in the lab indefinitely. So, the doctor took some. It is sometimes difficult to grapple with the reality that, like with so many issues of race, injustice, and reparation, we all collectively shrugged our shoulders and just kept using them in research. They were already so well established, the experiments have already been done, and after all, scientific integrity was on the line. I always tried to think of Henrietta when I fished a new orange-capped cryotube of HeLa cells out of the liquid nitrogen storage, holding her image in my mind and giving her space to breathe between the regimented steps of the thawing protocol, as her DNA warmed and came back to life.
Evolution
It is, of course, mutability that imparts the diversity of flora and fauna as described by Darwin: natural selection must have something to select. There is a delicate balance between accurate repair (maintenance of the status quo) and the opportunity for evolution (opposition to the static). And it is this mutability that stands defiant against the cold instinct to catalog, to fix, map, quantify, reduce. No matter the technologies that allow us to dictate to DNA, our attempts to dam and direct information flow, living archive becomes an oxymoron; preservation runs counter to living. Shades of Goldblum: life, uh… finds a way.
When Shipman and colleagues retrieve the image data from the bacterial colonies generations later, we see a visual representation of genetic imperfection: mixed-up pixels, blind spots, a flicker of absence in the horse’s hindquarters. Left long enough, an entire image archived in a living genome might eventually dissolve into unrecognizable static: we give the cells no selection advantage, no reason to keep the image safe and whole. (You can make a bacterial culture, but you can’t make them appreciate art.)
Science and photography both seek to contain nature in clean frames, affixed in order and stamped with external meaning. With the dissolution of the stored image, we begin to see how fixation may ultimately be futile. Like Muybridge’s motion, like a chromosomal rearrangement driving a new adaptation, life unfolds in the gaps between frames. As the gif dissolves, the anima of Annie G is freed, galloping into the spaces between things.
[1] Ed Yong, “Scientists Can Use CRISPR to Store Images and Movies in Bacteria,” Atlantic, July 12, 2017, https://www.theatlantic.com/science/archive/2017/07/scientists-can-use-crispr-to-store-images-and-movies-in-bacteria/533400/
[2] For a discussion of Eadweard Muybridge and the “shadow archive” of anthropological photography, see Shawn Michelle Smith’s At the Edge of Sight: Photography and the Unseen (Duke University Press, 2013).
[3] Lee Organic et al., “Random Access in Large-scale DNA Data Storage,” 36 (2018): 242-248, https://www.nature.com/articles/nbt.4079
[4] Aili Han and Daming Zhu, “A New DNA Encoding Method for Traveling Salesman Problem,” in Computational Intelligence and Bioinformatics, ICIC 2006, Lecture Notes in Computer Science, vol. 4115 (Springer: Berlin, Heidelberg), https://link.springer.com/chapter/10.1007/11816102_36
[5] Francis Crick, “The Double Helix: A Personal View,” Nature 248 (April 1974): 766-769, https://doi.org/10.1038/248766a0
Dilettante Mail
Get updates from us a few times a year.